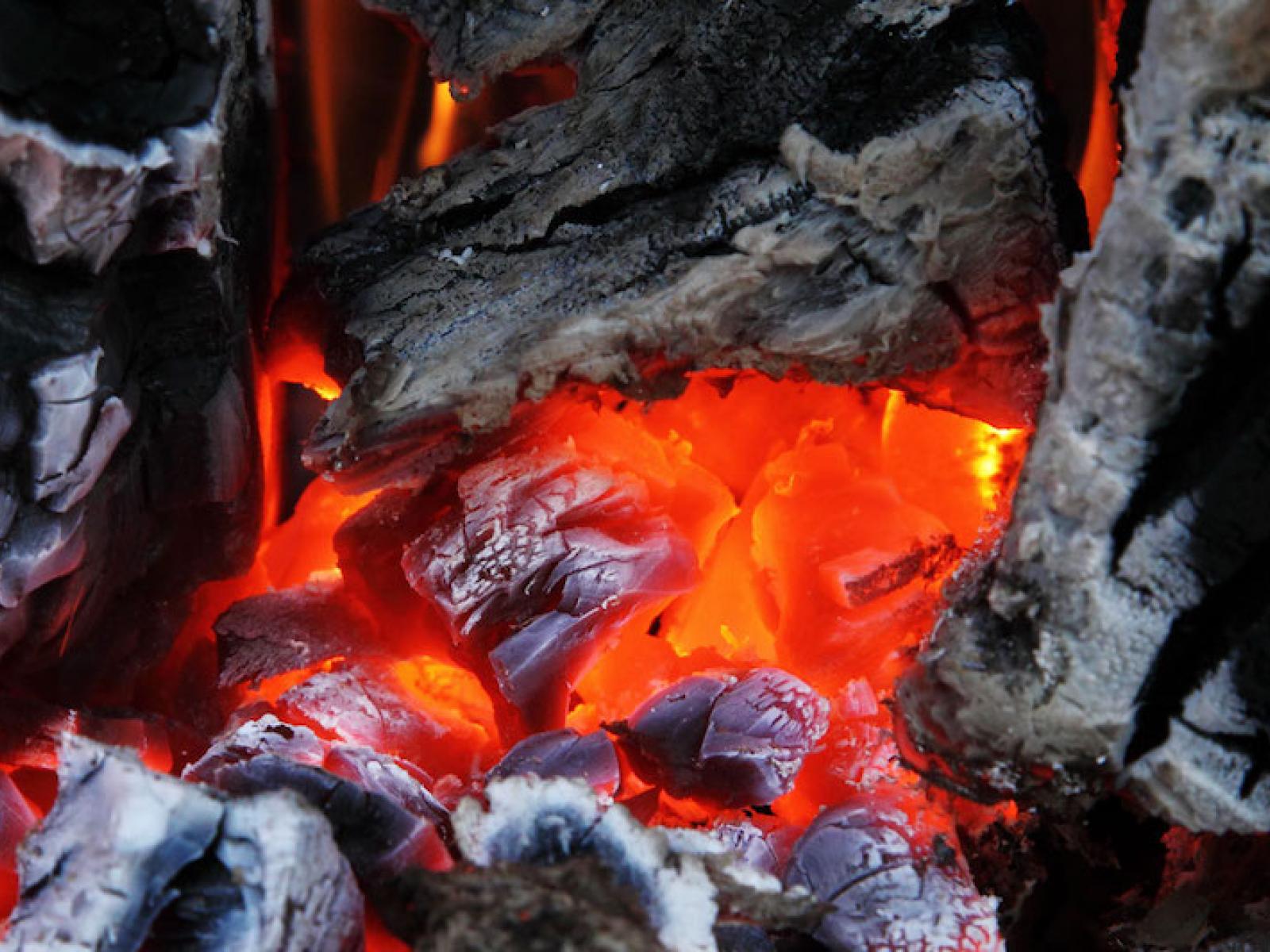
The basics of energy storage economics
Thursday, 18 June 2020
Green armchair experts see interseasonal energy storage as the technology that will bring their plans together, and think it is just a matter of investment and technological progress. Most sceptics also think it's about technology, but believe there are insuperable technical barriers. In reality, the main barrier is economic not technical. We explore this fundamental economic challenge with reference to a recent announcement regarding one of the more promising technologies.
Time after time, keyboard warriors treat energy storage as their deus ex machina to explain how we will get round the imbalances between energy demand and the production profile of their favoured technology. They explain airily that it's just a matter of investment. The technology (typically batteries) is coming on so fast that it will soon be economic for interseasonal storage.
Or if they hate renewables, they still treat long-term storage as a technical issue, but in that case an insuperable one.
See, for example, the replies to these tweets by Bishop Hill and Andrew Neil.
The reality is that the fundamental problem with energy storage for long periods (e.g. between seasons) is economic, not technical. Maybe there are resource limitations to build enough batteries, but there are other storage technologies that claim greater potential for scaling to this kind of use. Who knows what will be invented in future? But whatever the innovation, it will always face the following obstacle.
Above-ground compressed-air storage
A positive report about a British company deploying an innovative system for storage provides a useful jumping-off point to demonstrate the basic economics. To be clear, we take no view about the credibility of this technology. On the basis of its promoters' claims, it sounds promising. We take those claims broadly at face value here, as the problem has little to do with the technical feasibility. The problem remains even if advances like this are validated in deployment.
The report gives some basics:
- The scheme has capacity to store 250 MWh
- It costs £85m
- It will create 200 construction jobs and "a few dozen in the continuing operation" (is an employment cost of any less than £1,000,000 consistent with a few dozen employees in the UK?)
- It's expected to last 30-40 years
Naturally, there are a few omissions (no business will willingly reveal every detail of its economics). Most importantly, we have the energy capacity (MWh), but not the power capacity (MW). But they give us a clue that it can "power up to 200,000 homes for five hours", so presumably around 50 MW max output (although 250W sounds light for a home).
It would be useful to know if the maximum charging rate is the same as for discharge, but it's reasonable to assume that it won't be an order of magniture different.
The round-trip efficiency is a key value for any storage technology. The report does not tell us. This is widely understood to be a weakness of compressed-air storage. Existing systems are said to have an efficiency of around 60%, but it's believed that 70% is achievable with known technical advances. Perhaps they will go further in future. Highview cite 60-75% on their website. Let's take 70% as a generous figure at the moment.
The economics also depends on some aspects outside the control of the storage supplier. The most critical is the differential between the cost of the purchased electricity and the value of the discharged electricity. At present in the UK, the differential between low off-peak and peak prices is around 15p/kWh (£150/MWh) on a daily basis, and could be as much as 40p/kWh (£400/MWh) on longer-period differentials.
Calculating these differentials from retail prices is a generous assumption, as large storage systems will be playing in the wholesale market, whose prices are much lower. The differentials will probably also be somewhat lower, although the main difference between wholesale and retail is the smearing of the subsidy, distribution and system costs over all retail sales, which therefore should not greatly affect the relative values.
Let's take two extremes of use-cases to demonstrate the effect of usage on viability:
1. Smoothing the daily variations (365 charge/discharge cycles p.a.).
2. Interseasonal: storing summer excess for winter (1 charge/discharge cycle p.a.).
Daily smoothing
The system charges up every day during the cheapest off-peak periods in order to discharge every day during peak periods.
At 70% round-trip efficiency, they need to buy 357 MWh for each 250 MWh that they discharge. But they are averaging 5p/kWh for the 357 MWh (£17,850), while selling the 250 MWh for 20p/kWh (£50,000). That's £32,150 net revenue each day, or £11,734,750 p.a.
We know they have employment costs of at least £1,000,000, and their other operating costs will not be zero. Let's generously call it £10,000,000 EBITDA. That's an acceptable (though hardly exciting) IRR over 30-40 years of 11% on the initial £85,000,000 cost.
Interseasonal: storing summer excess for winter
The system charges in summer during the cheapest periods and discharges in winter during the peak periods (assuming they can be predicted).
They still need 357 MWh for each 250 MWh that they discharge. But in this scenario, that's the annual total as well.
The price differential is greater. They charge up at -5p/kWh (£-17,850) and they sell for 35p/kWh (£87,500). That's annual net revenue of £105,350.
On the above basis of operating costs and overheads of around £1.75m, they are losing £1.65m p.a. This is an obvious non-starter.
That should do you
It's as simple as that for those of you who get the maths. The return from energy storage is proportionate to the frequency of charge/discharge cycles. As most storage technologies have material capital and operating costs, they need to charge/discharge frequently (typically daily) to justify the investment. They can't do that and provide interseasonal storage.
What follows is a look at some of the nuances for those of you who think you are clever enough to spot an end-run round this fundamental mathematical constraint. You're actually dumber than the ones who can see that's it. I don't really care whether what follows persuades you or just wastes your time while you try to protect your delusions. You deserve it either way.
A middle ground?
OK. So they won't charge/discharge once a year. But perhaps there's a middle ground?
The challenge that we are told energy storage will solve is the seasonal/long-period imbalances between demand and the production profiles of intermittent generators. So there's no point considering a middle ground of (say) weekly rather than daily charge/discharge because that does nothing to address seasonality, nor does it match to the variations in production (and only modestly to demand variations). But it harms significantly the storage economics. That's pain without gain.
So what is the middle-ground use-profile that makes storage useful for long-period balancing without destroying the economic model of the storage facility?
There isn't one. Interseasonal storage is fundamentally about storing up the energy in one season (typically summer) and releasing it in another (typically winter). If you try to squeeze in extra charge-discharge cycles, you help the project economics but you remove the interseasonal nature of the storage.
For example, there's no such thing as charging up in June for discharge in January but somehow also charging in April for discharge in October. If you've charged in April intending to discharge in October, you can't also charge in June to discharge in January because when you come to charge in June, you're already full from charging in April. You have to do something like charge in June for discharge in November and then charge in December for discharge in February. That's no longer interseasonal to a meaningful extent, and certainly not more so than simply charging in summer and discharging in winter.
That's pretty much the fiction of interseasonal storage for solar disposed of. In temperate regions, solar is very seasonal. Output in winter is minimal. If we want to balance this with energy storage rather than dispatchable generation, we literally have to have storage systems to charge in summer and discharge in winter.
Smoothing wind
The least-bad middle-ground application could be smoothing the non-seasonal intermittency of wind power. The economics of this cannot be simplified as above, because the production profile is largely stochastic with minimal cyclical elements. In other words, sometimes the wind blows strongly for sustained periods (even a week or more), sometimes we are becalmed for sustained periods, and at other times, the variation is more frequent, but never regular.
Imagine a storage facility trying to "flatten that curve". If it's sized so it can charge up for 7 days before discharging for 7 days so it can smooth out the longer-period variation, most of the capacity will be under-utilised for the short-period variations. If it's sized to the shorter-period variation, then it won't have the capacity to smooth the longer-period variations. Sizing to the shorter-period variations will be more economic, but this does nothing to address the impact of sustained cyclonic and anti-cyclonic periods on security of supply, let alone to match intermittent output to the cyclical variations in demand.
Instead of a simple calculation as above, you have to model the production and demand profiles and balancing-market prices, and adjust for reasonable expectations of future developments, to judge the potential and cost of this type of storage application. Our Future Scenarios model allows you to do exactly that. The outcome, inevitably, is that the first few GWh of storage capacity can focus on the smaller, more-frequent variations and perhaps do OK. But as you add capacity, the opportunities are increasingly covered in the short-period variations, and the extra capacity has to try to survive on the longer-period variations, which destroys their economics.
You end up with a marginal-utilisation curve like this. This is the output from the model where we have assumed that we have followed the government's plans for massive expansion of offshore wind and solar, and rather than rely on coal or gas for balancing, we have deployed a huge amount of compressed-air storage (2,000 GWh, 10 GW) to try to smooth out the imbalances. It also assumes material progress on the government's plans to electrify heat (40% of total heat, all heat pumps where new) and transport (about 50% of road transport).
The marginal-utilisation curve breaks the storage capacity into chunks of 100 GWh and shows how each additional chunk is used somewhat less as the gaps for it to fill are increasingly filled by the previous chunks. This would manifest itself as increasing costs of storage as capacity is added, becoming prohibitive in this case around 1,100 GWh.
This is massive hypothetical investment in energy storage. If 250 MWh / 50 MW costs £85m, then the capital cost of 2,000 GWh / 10 GW would be around £100bn, before any learning-curve savings.1 Yet it's not enough (as the "Electricity supply margin" chart shows) to store the wind when it blows and the sun when it shines to ensure electricity is available to meet demand at all times. This would be exacerbated if only the more feasible half of this storage capacity were installed.
And this is in a world where we are nowhere near full decarbonisation yet. This might be just about on-plan for the government's Net-Zero strategy for 2030.
Feasible long-period energy storage technologies
There are only two technologies known to be feasible for such long-period storage:
1. Hydro (conventional dam across a valley, not pumped-storage or run-of-river)
2. Biomass
Funny enough, both of these technologies are effectively solar energy. They may not be as efficient as photovoltaics, but their capital- and operating-costs make them uniquely suitable for long-term storage. Contrary to myth, efficiency is far from being all-important.
Hydro can effectively hold back the rain that falls between (say) April and November for release as required from December to March. It's solar because it is harnessing the water cycle driven by solar evaporation. Its operating costs are so low and its potential scale so large that it can hold back meaningful quantities of energy for winter at modest marginal cost, although it's a long payback.
Biomass can be stored almost for free: cut it when suitable (depending on your weather/ground-conditions) and leave at roadside (drying to some extent) for collection as required. It's solar because it is harnessing the power of photosynthesis. The economics of biomass for seasonal storage depend on the cost of the technology in which it is used, as well as the fuel, but the fuel itself is eminently suitable for seasonal deployment.
For instance, new biomass power may be expensive to run seasonally as it needs to recover its capital cost and has non-negligible fixed and operating costs. But using biomass in fully-depreciated generating plant or for heat (where all the competition is also seasonal) may be viable, although investors may still prefer to run continuously regardless of demand if the support mechanisms are structured badly (as they are in the UK).
It is unlikely that other technologies will match these two for long-period storage. Not because of technology, but because of economics. They have greater capital and operating costs that (as illustrated above) cannot be recovered from infrequent use.
That makes the interseasonal storage of intermittent electricity highly problematic, to put it diplomatically. Neither hydro nor biomass is very suitable. It needs a technology that takes in electricity then releases it as required. But they are too expensive for long-period storage. You can time-shift solar from day to evening with chemical or mechanical storage technologies, but you can't time-shift it from summer to winter. The best you can do is find uses that match the seasonal profile, and resort to other technologies to fill in the gaps in the intermittent output.
The least bad option we have come up with so far is exporting to countries with large hydro potential (that means Norway within reasonable range of the UK) so they use our electricity when we've got too much, and save their hydro (for their own use and to export to us) for when we don't have enough. But Norway is a small country compared to us, in terms of economy and population. And we are not the only ones wanting to do this. The Danes and Germans are already doing it, to a problematic extent in the latter case. We can't all piggyback on Norway, like a herd of elephants piggybacking on a mouse. And we can't build our own because we don't have the geography or the political will for it.
Not nuclear
Certain armchair warriors like to cite nuclear as the leading contender to fill in those gaps. It can be done but it makes no sense. Technically, you can ramp nuclear down and up if it doesn't have to be too responsive. But what is the point? You save almost no cost or carbon when it is ramped down. What purpose are the intermittents serving that force nuclear power stations to be ramped down and up, versus simply running the nuclear more continuously and not bothering with the intermittents at all?
Not compressed-air storage (at the moment)
Let's be clear what Highview mean by long duration storage. The announced project has storage capacity for 5 hours at full power. In other case studies, they assume 10 hours.
This is not "long duration" in the sense of seasonal storage, nor even long enough for the longer-period swings in wind output. They mean "long duration" in the sense of significantly longer than the applications at which batteries are primarily targeted. It's "long duration" in the same sense as pumped storage, which looks like their target competitor: the ability to run for several sustained hours within a daily charge/discharge cycle.
Sure, some of the reporting also talked about their ability to store the electricity "for weeks". In that case, I have a bucket that is equally advanced. Water can be filled and discharged fast or slow, and if it's not discharged after filling, it can hold water "for weeks" or longer.
This is not a serious claim to long duration. Taken at face value, it refers to the potential for expensive equipment to stand idle, earning nothing, "for weeks".
The marginal cost of this technology's storage capacity is already relatively cheap, as opposed to the generating potential, which is expensive. The hope for this technology is that it can be further reduced so that it is cheap to expand the storage relative to the power, to extend the charge/discharge period. The problem is the same one described above. Extending the storage capacity not only increases the cost (however marginally); it also reduces the number of charge/discharge cycles in a year by extending the duration of each cycle. It's a fundamental, immovable obstacle, to which the only solution is very low capital and operating cost.
Highview are focused on projects with 5-10 hours of storage capacity not because it's a technical constraint, but because it's the economically-optimal capacity for their technology, even though an advantage of their technology is the relatively-low cost of storage capacity.
Not cost-saving
Let's say someone came up with a storage technology that was economic at the current differential between peak and off-peak prices. That may help our balancing problem. It is unlikely to help the economics of wind and solar, and therefore reduce the cost to consumers. It makes its money from the differential. If the differential comes down, the storage ceases to be economic. If the differential remains high, that reflects low electricity prices when the intermittents produce most of their output, and high prices when they aren't producing much. It's a non-existent solution that wouldn't save cost even if it did exist.
Fundamentally, you have to match supply to demand. If you can't produce when it's needed, there will be significant costs to align supply to demand. These are indirect costs of intermittency. They are ignored by proponents of intermittents, and are invisible in the wholesale prices those people use to pretend intermittents are cheap (read "not worth much"). But they have to be covered and end up one way or another on consumers' or taxpayers' bills.
Hence the widening divergence in the UK between a falling wholesale price and an increasing retail price. In the mid-2000s, the retail price was around 60% more than the wholesale price. Now the retail price is over 200% more than the wholesale price. Think of that next time a wind or solar advocate tells you how cheap they are.
1. The marginal cost of storage capacity is given as $50/kWh at https://www.highviewpower.com/wp-content/uploads/2019/11/ESA-storage-exchange-poster-2019-2.pdf. That's around £40/kWh. That puts the cost of the storage element of the announced project at around £10,000,000, leaving £75,000,000 for the generation/charging element. At 50 MW, that puts the cost of this element at £1,500/kW. We apply these ratios to 2,000 GWh / 10 GW to get a cost of £95bn. These ratios are consistent with the advertised advantage of this technology, that the marginal cost of additional storage is relatively low, which is why they are focused on longer storage opportunities.
Related blogs
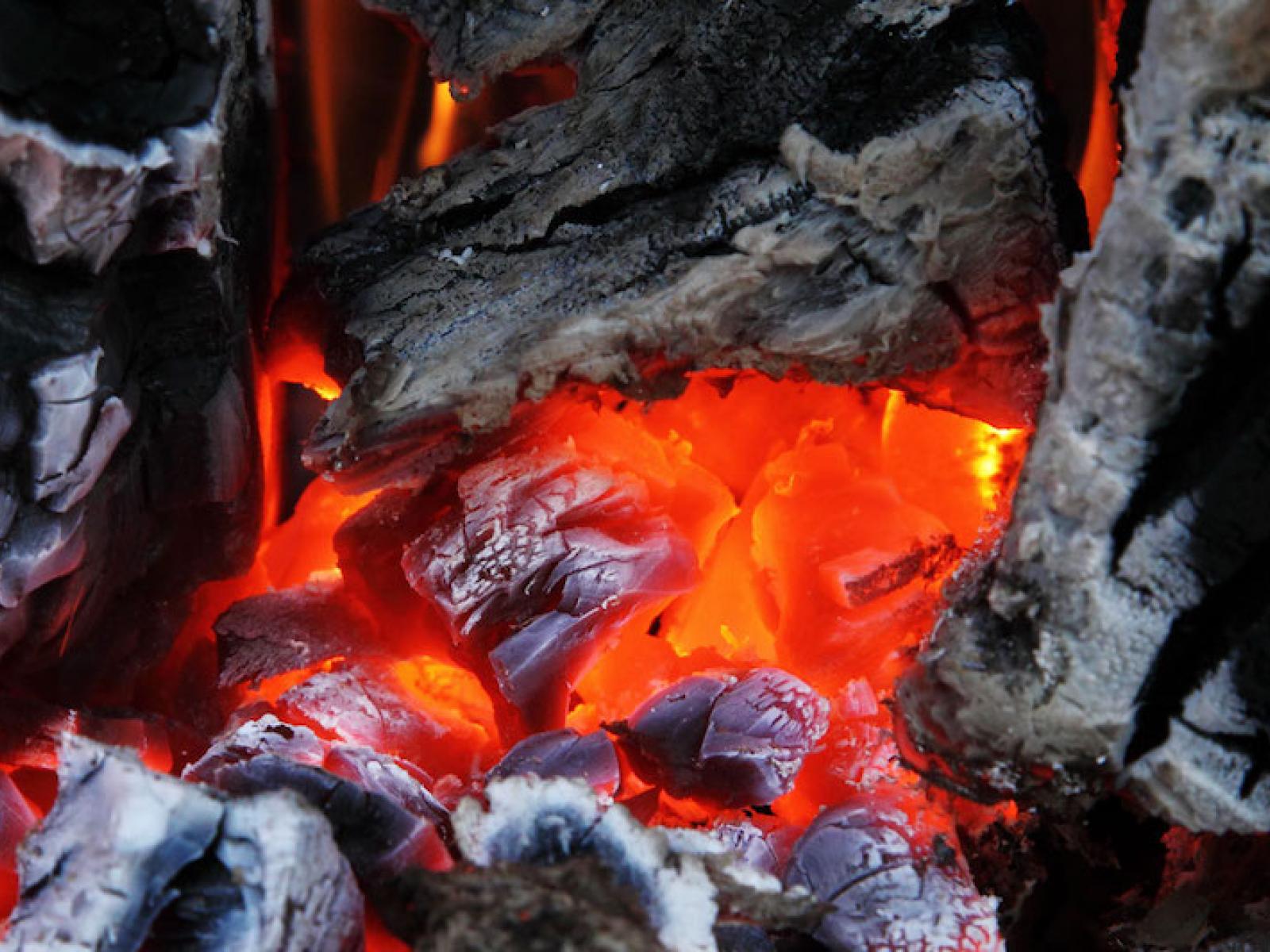
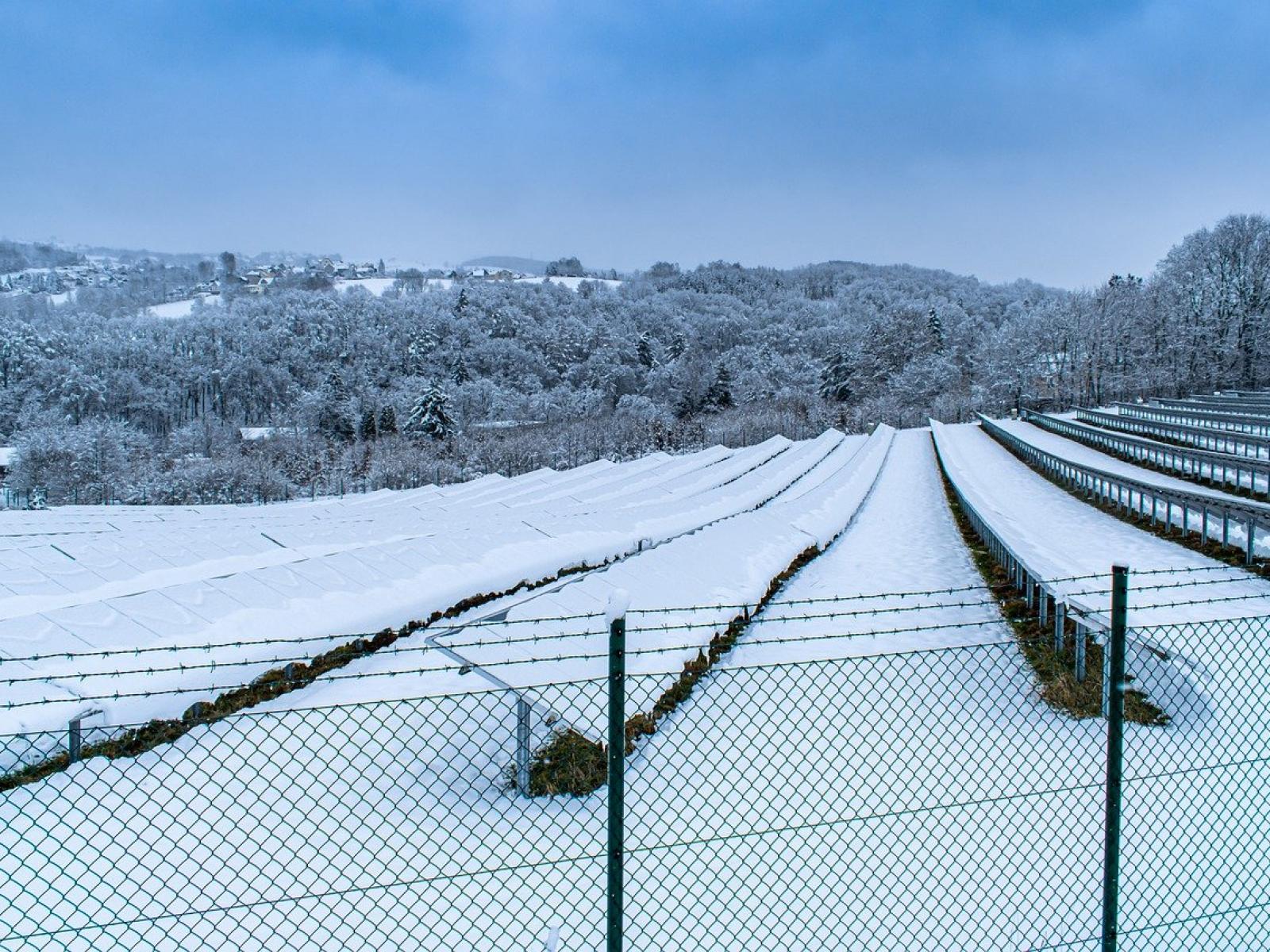
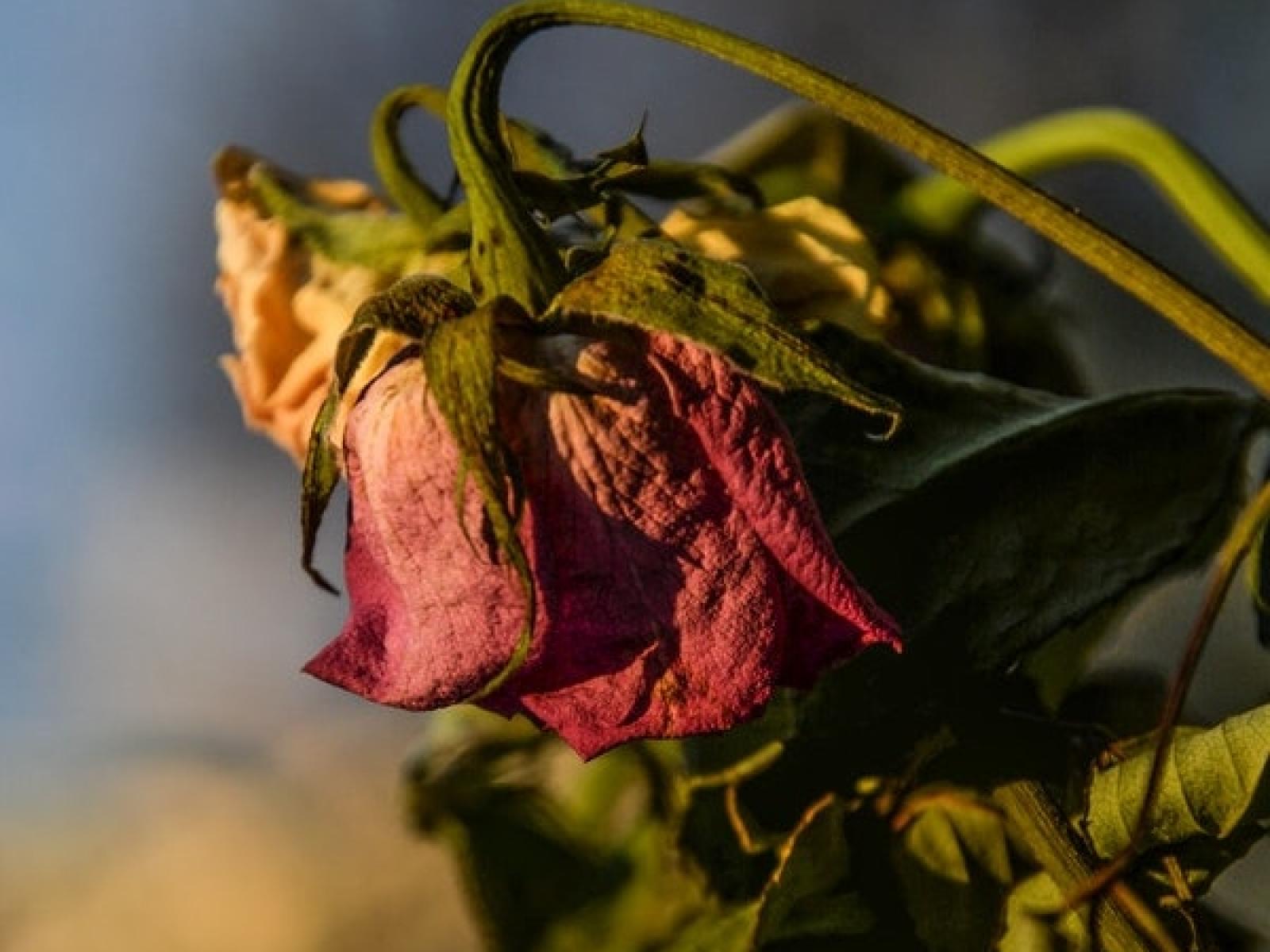